A solar eclipse is a magnificent natural phenomenon, during which the direct Sun is shadowed by the moon to darken the daytime sky (Vollmer, Shaw, 2018). In this article, I would like to draw your attention to another phenomenon, which I “discovered” accidentally during the Great American Eclipse – the visual range changes.
1. INTRODUCTION
I will say again, that normally an overwhelming majority of observers fascinate the solar corona during the eclipse. However, it’s good to know, that there are also interesting optical phenomena away from the Sun. This is, for instance, the angular distribution of both sky brightness and polarization change dramatically and abruptly at the onset of the end of the totality. Another one is the sky color and illumination changes, which I have shown in this article. In addition, the totality sky is sufficiently dark to allow naked-eye observations of stars and planets of approximately 3.5 Magnitude or higher brightness, yet diffraction coronas and halos may remain visible (although visible rainbows are very unlikely)(Konnen, Hinz, 2009).
The interesting subject is also visibility change during the solar eclipse. The visual range is an important thing for mountaineers, who desire to watch the mountains or different objects closely or further located, and for people admiring long-distance observations. The visual range changes during the solar eclipse is a very interesting issue because the lack of direct solar illumination of part or all of the path between an observer and a distant object can result in whether that object will be visible or not. This is the thing, that I address in this article. Having a decent knowledge about the extension of visual range during total solar eclipse observers and mountaineers will be able to plan their eclipse observations in mountainous areas. My observation and support materials come from the Great American Eclipse, which was observed in the mountainous-looking roughness of the western part of the USA.
2. THE OBSERVATION METHODS AND LOCATION
A basic observation method of the visual range extension is pointing the camera toward the presumed or barely visible remote mountain range. The observations carried out during the last totality were planned rather spontaneously. In order to continue these observations during forthcoming total solar eclipses the most helpful will be the Urlich Deuschle panorama generator or alternatively the PeakFinder website. Using these panorama generators you are able to set your observation place both against the umbral path and mountain ranges located at certain azimuth with a rough distance to them. Another helpful tool is the Google Earth path measure and estimated real-time shadow presence, which you can make on your own. I have prepared 2 examples for the forthcoming 2 South American eclipses, which you can watch below.
An important part of the observation method is also analyzing the footage, where you can see the details, snap the view, and edit it giving a result beyond the ability of human vision.
For the explanation of the extension visual range during the Great American Eclipse, I used the data from 2 observation places. One was mine, on U.S. Hwy 26 between Riverton and Shoshoni. There is a view towards Owl Creek Mt mainly and other remote mountains occasionally (especially the Wind River Range during the totality). The Owl Creek Mts were at around 30 – 40 km distance (Arrowhead Ridge, Copper Mountain) and some of them are more distant (Pic. 1). A western part of the Owl Creek Mts with Owl Creek Mountain, located about 60-65 km from my observation site. The highest mountain range possible to see – the Wind River Range (which has been considered in the preparation for the eclipse in order to see fleeting lunar shadow) was at about 90-100km distance from my observation venue.
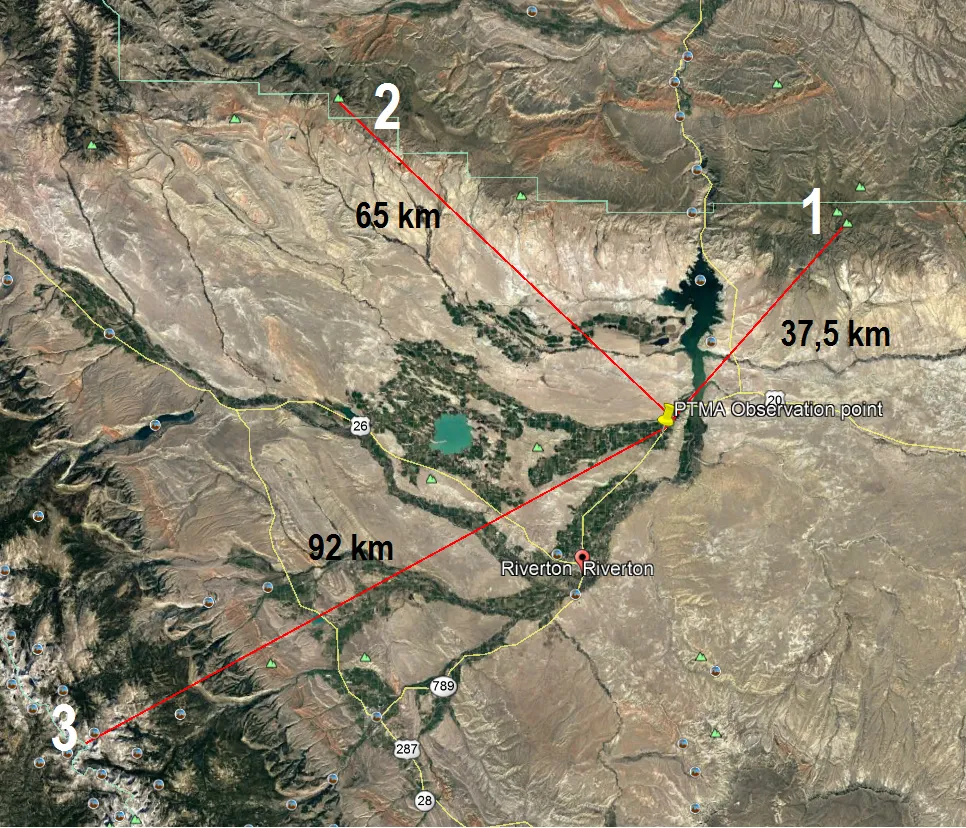
For another observation place considered, I used Carsten’s Jonas footage with the camera headed southeast. Carsten Jonas was observing the solar eclipse from Round Butte Overlook Park near Madras. There are some mountains at a similar distance: The Grizzly Mountain – 31km and the Gray Butte – 25 km.
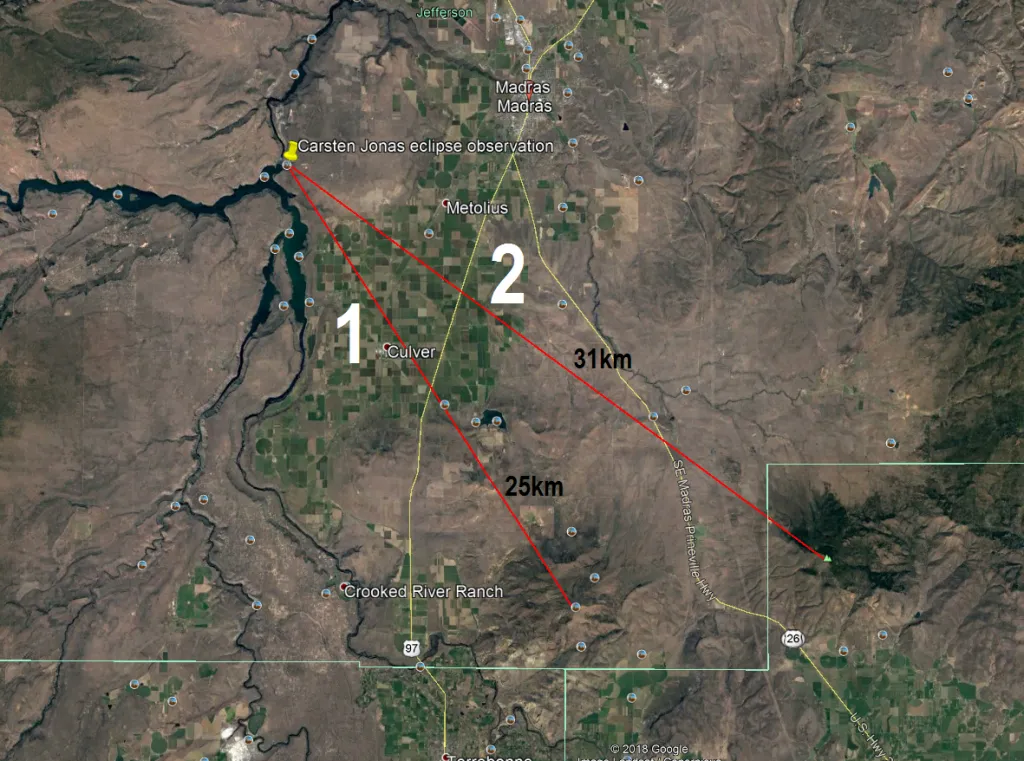
Both observation places have a common denominator: they are located almost in the center of the totality path (first place with shadow deep 99,5% and 2m23s totality, second place was a little bit further with shadow deep 75,5% and 2m00s totality. Due to this all remote objects covered by the camera frame experienced a shorter period of totality. The thing, that differs between both these places is direction. I was capturing mountains in the northwest and north direction, whereas Carsten Jonas did it toward the southeast. There is also another thing, which varies our places so far – this is a haze concentration. These aerosols were omnipresent, however, Madras experienced extremely high concentrations on eclipse day. It is reflected in the mountain view. The Grizzly Mountain was almost invisible throughout the partial phase, whilst the Owl Creek Mts were only a bit veiled by haze. The solar position was 53 deg above the SE horizon (azimuth 139 deg) in my observation place and 42 deg above the ESE horizon (azimuth 119 deg).
In my observation, I used the Nikon D5300 camera with a Nikkor 55-300mm lens and also an Olympus E-510 camera with a kit lens 14-42mm. Carsten Jonas used Canon 7D2. In order to outreach my survey, I used also drone-perspective footage made by Mateusz Windak. He was observing the totality also near Madras, but from the other side – on NE (Quaale Rd), 16 km northeast of Madras. During a whole totality, the drone camera was headed toward the NNE direction. Unlike Carsten’s Jonas footage haze density appeared to be much lower, possibly due to the higher location of the observation point plus additional drone altitude. Due to this the visibility range was much more extended.
3. VISUAL RANGE IN DAYLIGHT CONDITIONS
A simplified model for visual ranges in daylight conditions has been discussed in previous articles, where I fairly listed most of the factors, that influence on it. Here I would like to extend this topic a bit in terms of the comparison to the solar eclipse conditions.
When we are looking at a distant object our eye detects both scattered light from the object as well as airlight containing i.e. scattered radiation from the molecules along the line of sight. In particular, the visual range depends on the radiance difference between the object and the adjacent horizon sky (which only consists of airlight)(Vollmer, Shaw, 2018).
The Earth’s atmosphere is characterized by multiple scattering as well as ground reflection scattered into the line of sight. It means, that basically there is no sharp transition at the shadow edge produced by thick clouds and also the Moon during the totality. The problems with cloudiness impact on solar irradiation have been discussed in the previous article, however, I took into account a sharp transition at the shadow edge for the sake of simplification.
Every light scattered in the atmosphere is undermined by haze presence as well as molecular and aerosol absorption. Most of the paths through the atmosphere to remote objects are slightly oblique if the observer and object have different heights. Due to this the path between an observer and the object observed is a bit longer, therefore a thicker haze concentration appears on this line of sight. However, we can omit this issue, because, on the other hand, the atmosphere at the mountain top level is a bit clearer than inside the surface layer. This difference in atmospheric clarity between these two levels is enough to make the mountain tops better visible than their bases, which has been described previously. Moreover, the molecules and aerosols being in the line of sight have a different incident sun radiance, especially when the path from the observer to an object is different due to different solar positions in the sky. It plays a huge role especially near sunrise and sunset, when a single angular degree changes the vista’s appearance significantly.
When referring to all these factors, raised both above and in previous writing to visibility range on eclipse day we have to take into account also the aerosol optical thickness, which was different both for mine and Carsten’s Jonas observation point. The aerosol optical depth issue has been explained here.
4. MODELING THE VISUAL RANGE FOR INHOMOGENEOUS ILLUMINATION OF A LINE OF SIGHTS.
Based on my own observations of moving lunar shadows across the mountain ranges as well as the discussion of Vollmer and Shaw I can say, that there is one major factor, which makes the inhomogeneous illumination of the line of sight. This is obviously a lunar shadow placed along the line of sight. Inhomogeneous irradiation, as I mentioned before must be responsible for the increase in the visual range.
There are six different variations of radiance along the line of sight (Vollmer, Shaw, 2018), however, I will write about them a bit later. During my amateur observation of shadow movement I could spot a basic 4 variations of radiance in terms of the particular mountain range (Owl Creek Mts) observed during the total solar eclipse.
A. HOMOGENEOUS IRRADIATION AT NORMAL CONDITIONS UNDER CLEAR SKY – where there is no difference in illumination alongside the line of sight (Pic. 3). This situation is typical once the sky is clear or veiled by thin clouds. In this case, the visibility range is the smallest due to incident light scattering by haze or molecules.
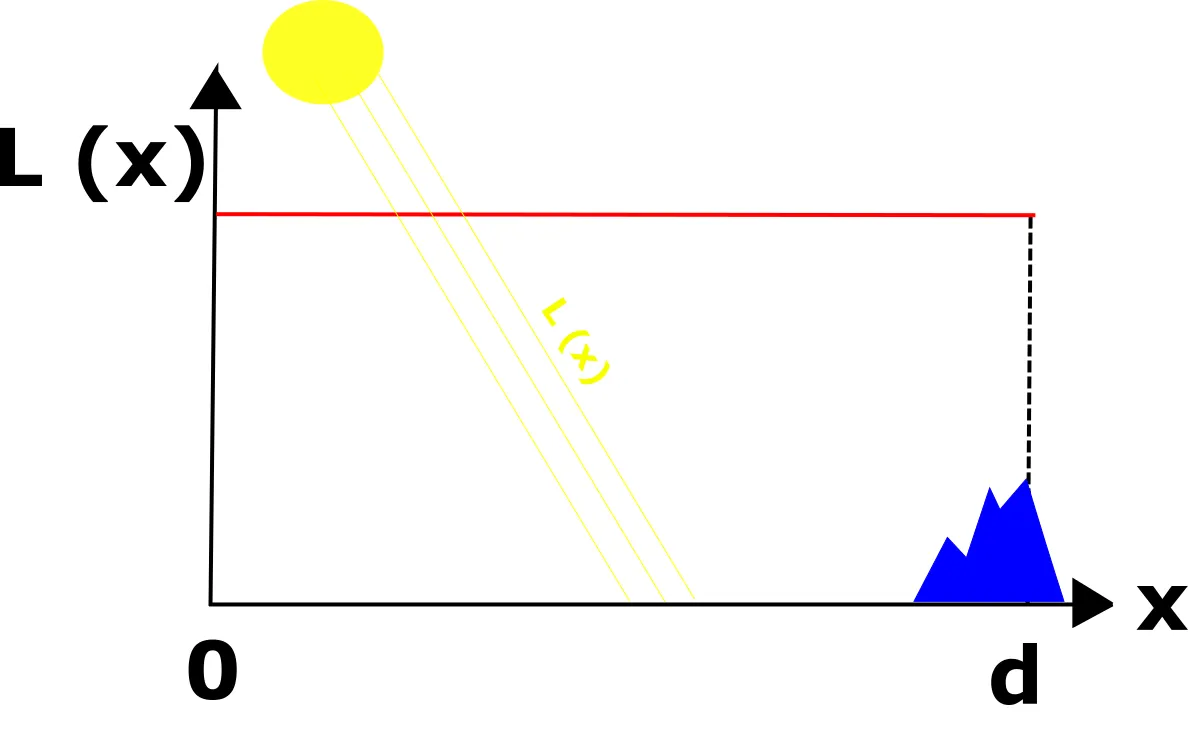
B. HOMOGENEOUS IRRADIATION UNDER THE TOTAL SOLAR ECLIPSE CONDITIONS – the analogous situation, where the irradiation profile is the same alongside a whole line of sight. This situation occurs when both an observer and the distant object observed are inside the lunar shadow. As I wrote in the previous section a similar situation can happen under a thick cloud, but usually on a smaller scale. In this case, the visibility is the best and the visual range is the longest. Neither haze nor molecules don’t scatter sunlight only the light diffused in the Earth’s atmosphere. However, this is the time, when these particles start to absorb the light. As a result under hazy conditions, the contrast between a remote object and the lower part of the sky is reduced.
We must bear in mind, that the situation described has been simplified. The light level changes inside the umbra too, which I will describe in the next article. Usually, the darkest moment occurs around the mid-eclipse, but not roughly at the mid-eclipse moment, as shown in the image below for the sake of simplification (Pic. 4).
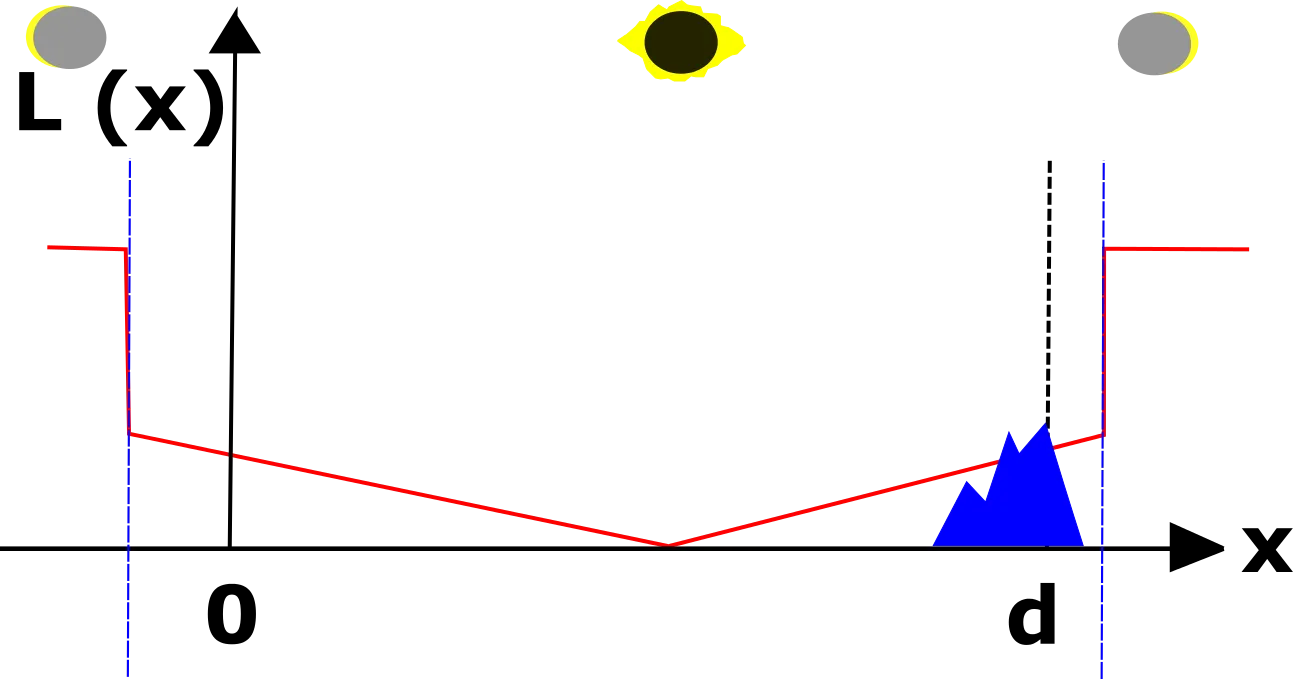
Because the umbral region is restricted (max. to about 270km, when the eclipse occurs around midday), always the sky above the horizon beyond is much brighter, making a perfect contrast with shaded objects or mountains (Pic. 4). They appear to be black against the illuminated sky beyond. The high contrast ratio makes them visible, even when a haze concentration is big, although any haze presence leads to attenuating the diffuse radiated light from the illuminated sky outside the umbra.
A bright sky affects the shaded region, making it slightly more illuminated near the umbral edges (usually up to 20-25s after 2nd and before 3rd contact), however not bright enough to decrease the contrast ratio with illuminated sky beyond. We can see this illumination difference, which is expressed by a thick haze located towards the umbral edge, due to its forward scattering (Pic. 5, 6). This issue will be explained more in the next article.
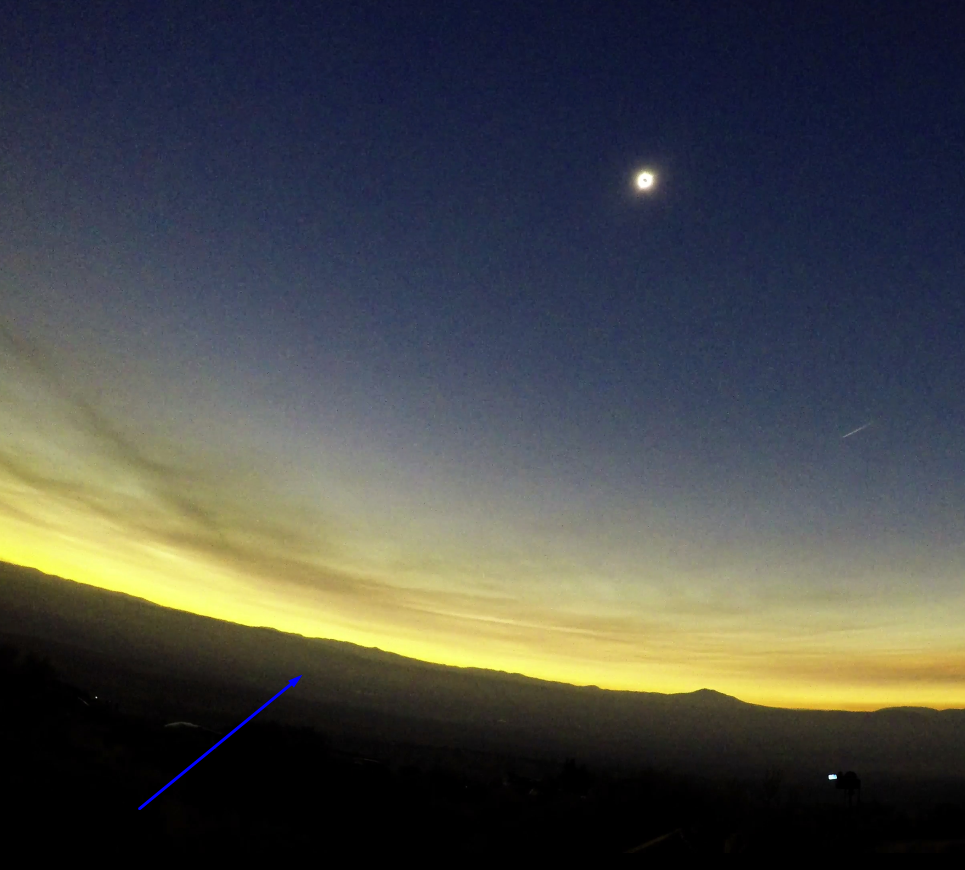
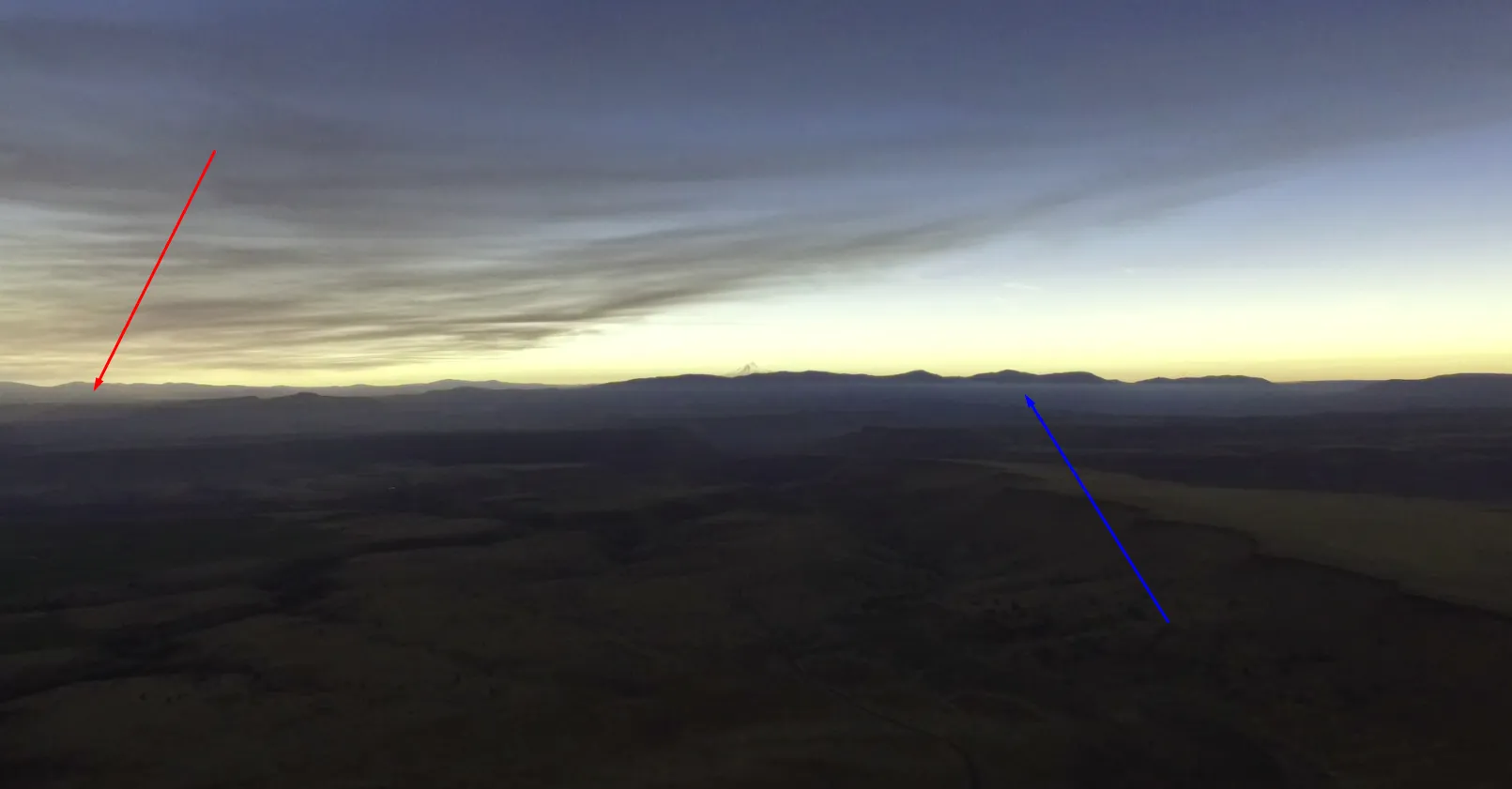
C. HETEROGENEOUS IRRADIATION WHEN OBSERVER IS ILLUMINATED, BUT A DISTANT OBJECT SHADED – a classic situation from forthcoming totality when fainting sun crescent still illuminates the area near the observer but objects located further towards shadow-in are already inside the umbra. Usually, we can see it just before the totality approaches. Depending on the visual range and distance to the object observed it can be at around 98% obscuration upwards (less than 1m50s). In Great American Eclipse conditions, the umbral diameter was around 110 km. A crescent sun obscured in 98% was around 100 km from the edge of totality. From this moment the C-model (Pic. 7, 8) was observed. I think, for each eclipse, the best moment to see this situation is the tens of seconds before totality. This model can be also implemented in deep partial eclipse situations when the fleeting shadow is seen from a distance less than the visibility range. Then some remote objects finally can be merged with the darkening sky. We also must know, that this model doesn’t work when the umbra approaches closer, because as the illuminated space shrinks, the darker side is revealed with a bright horizon sky at the opposite side (Pic. 7) of the umbra, unless is overcast, about which a bit later in this article.
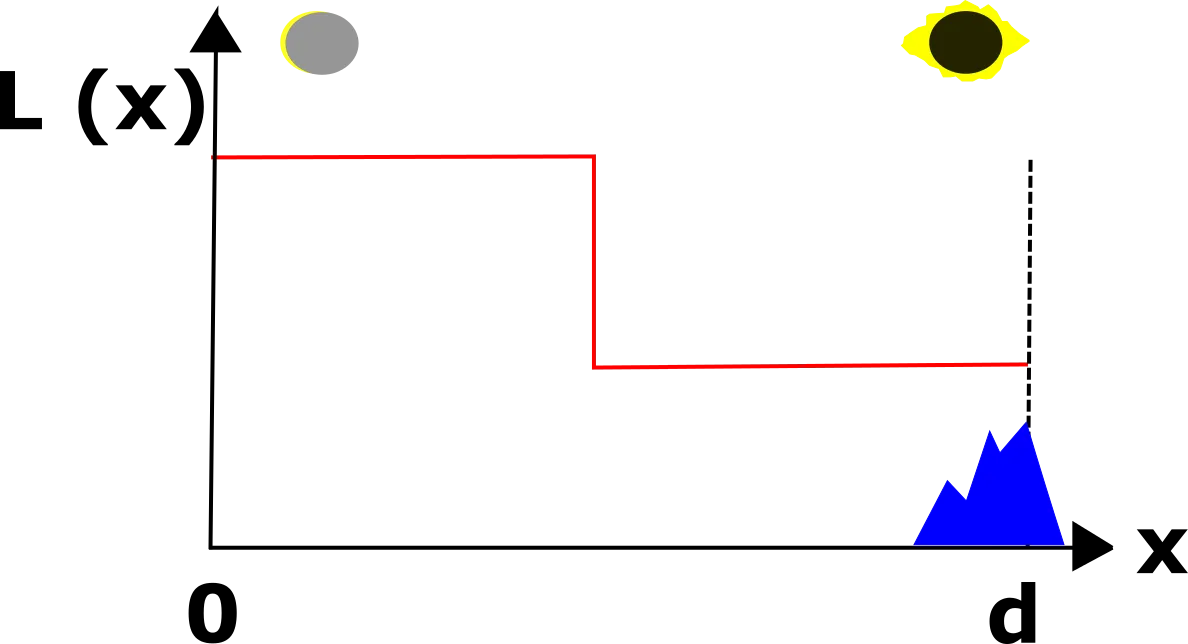
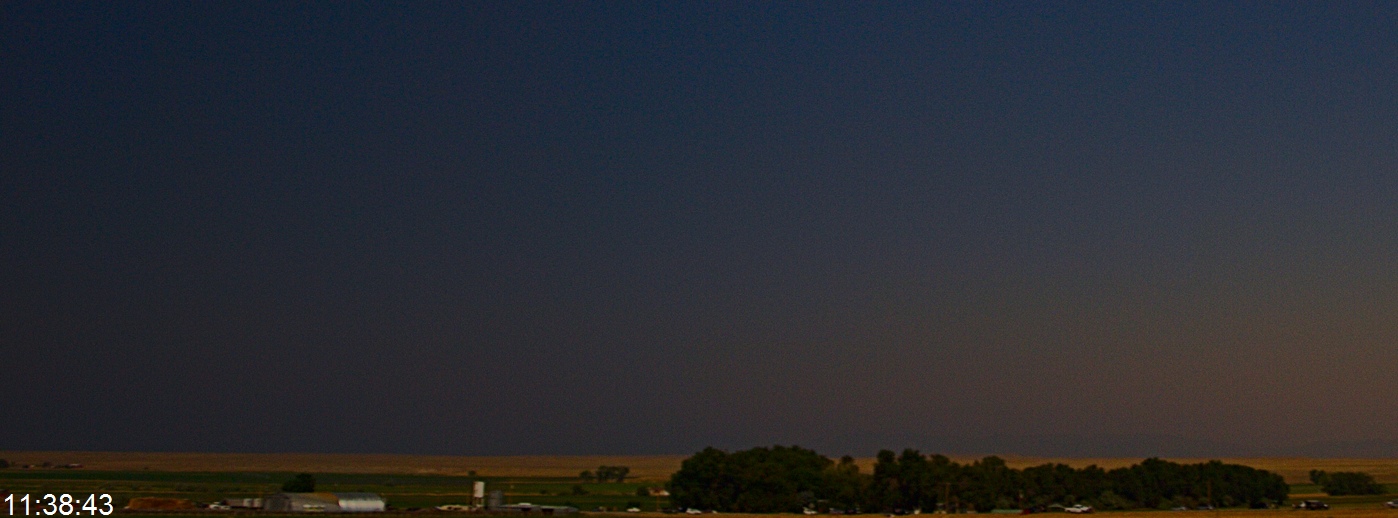
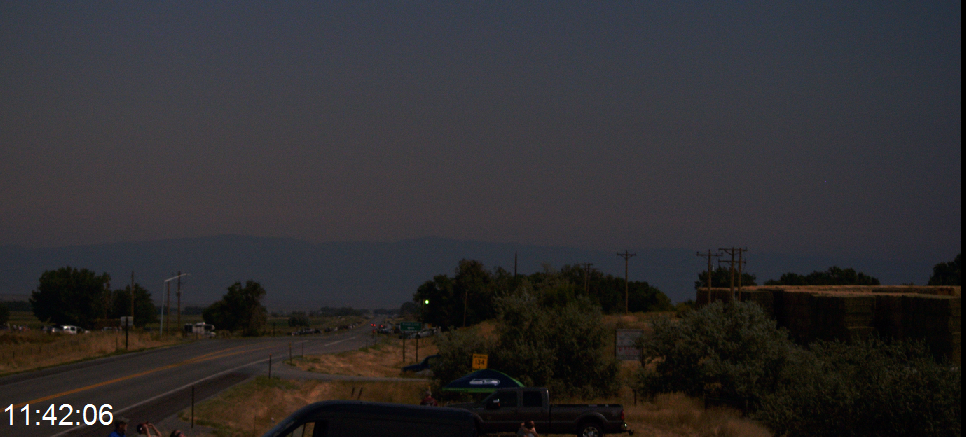
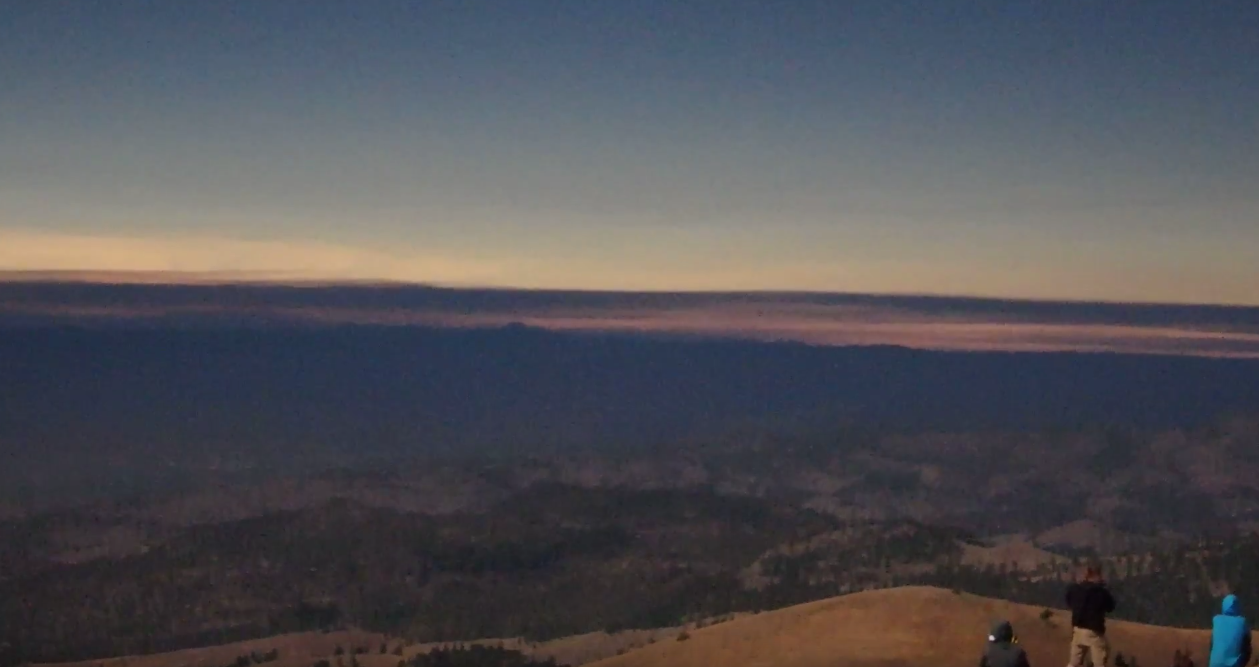
D. HETEROGENEOUS IRRADIATION WHEN OBSERVER IS SHADED AND REMOTE OBJECT ILLUMINATED – this is an invert situation, where an observer is plunged in totality but the remote objects observed by him are already (or yet) illuminated by the crescent sun. The D-model (Pic. 11) is a common denominator for all observations taken into account because in all cases the umbral depth of the observation place was bigger than the remote objects observed. To simplify it, the observer, in all cases was located close to the middle of the totality path, whereas all objects observed were more outside, closer to the path edge. It means, that in all cases totality lasted longer in the observation place than near the objects observed. For example, in my observation place, the totality lasted 2m23s, whilst at the Owl Creek Mts range just from 1m50s to 2m03s only. It means, that when the line of sight coincided with exactly the same moment of the greatest eclipse the observer could experience the D-model twice (Pic. 12). Both just after 2nd contact, where the remote object was illuminated for several seconds and shortly before 3rd contact when the same remote object was already illuminated by the increasing sun. An analogous situation could have happened for someone, who decided to watch the eclipse near the edge of totality. In that case yet or already illuminated observer would be able to see shaded remote mountains twice because the C-model works then (Pic. 7-10). A visual effect of the D-model is a strong contrast with the dark ground, brighter object, and much brighter sky (Pic. 12). Sometimes an observer distinguishes some relief details on the remote object, which depends on the haze concentration. Receding the umbra from the mountain is expressed by changing color from black-looking to more yellowish likewise in the example provided (Pic. 12).
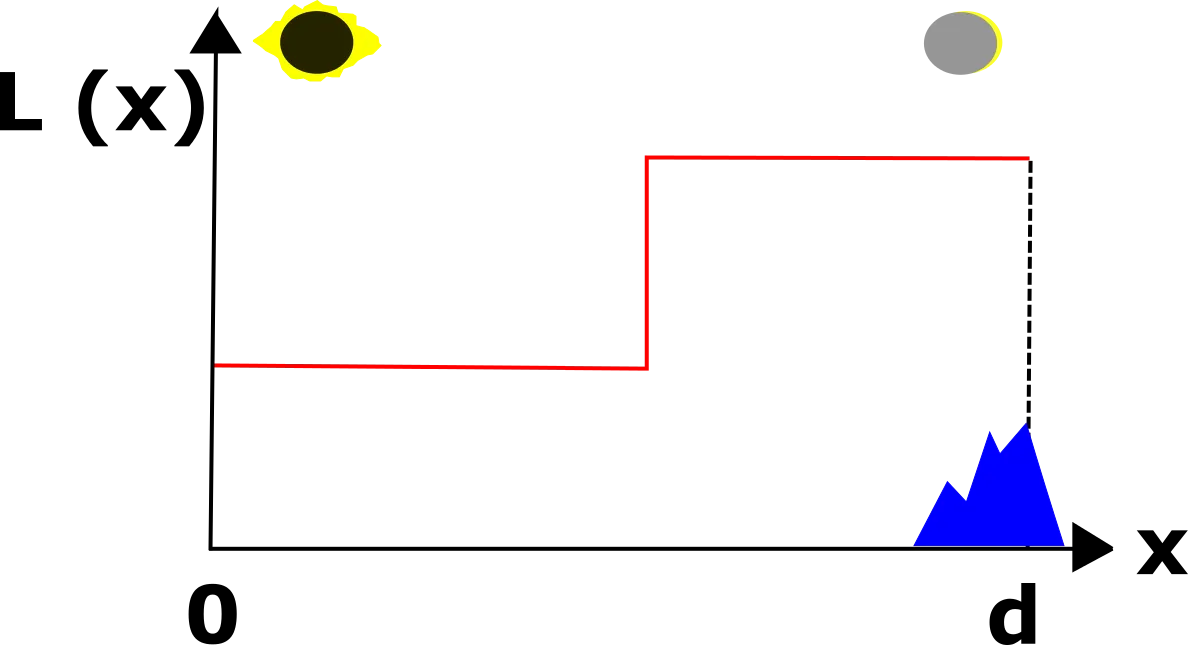
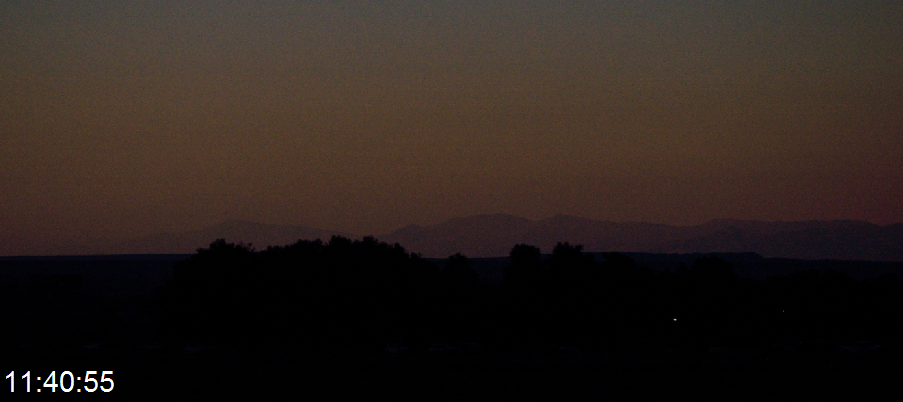
E. HETEROGENEOUS IRRADIATION CAUSED PRENUMBRAL IMPACT BEFORE THE ECLIPSE – This is the model that covers a nonuniformity of light alongside the line of sight caused by penumbral impact. This model assumes that illumination in the observation place is bigger than near the object observed. It works through a whole partial phase, however, the human eye cannot detect this difference until 86-88% obscuration, when the umbra becomes present in the sky (under clear sky weather towards the direction, where forward scattering plays the main role). When the sky becomes significantly darker, especially near the horizon the distant objects, being less illuminated can be washed out of the greyish-blue hue of the sky. This situation is more visible as the eclipse progresses covering the Sun up to the moment when the observed remote object is plunged into the umbra. The E-model of heterogeneous irradiation causes additional visibility impairment towards the shadow-in direction (Pic. 13, 14), but the reduction of light scattering ability by particles suspended on the line of sight is an advantage for the observer.
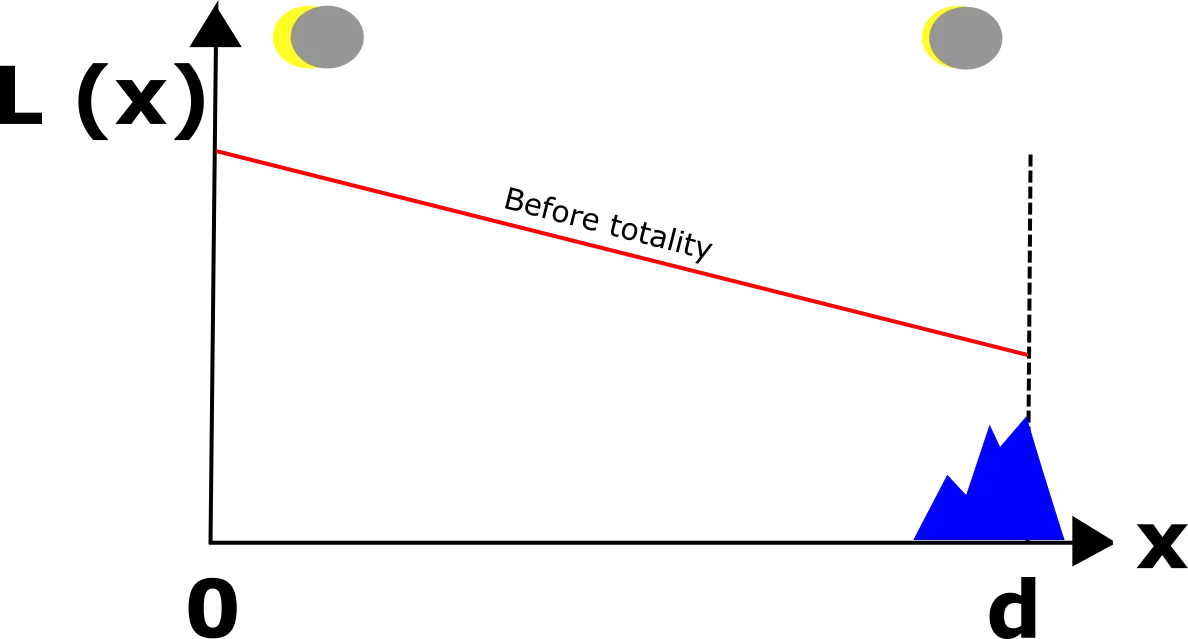
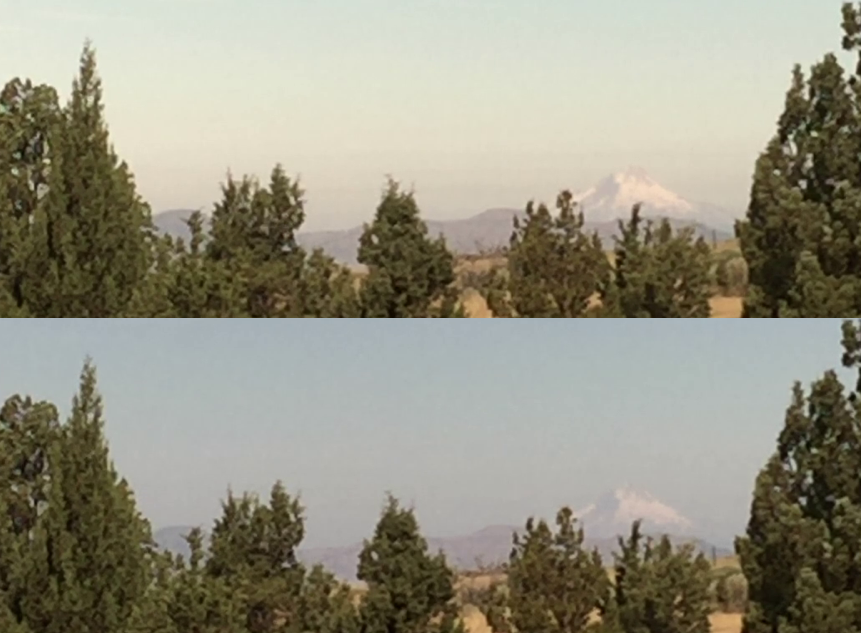
When our distant object is located towards the umbral position, then is much harder to spot him.
F. HETEROGENEOUS IRRADIATION CAUSED PRENUMBRAL IMPACT AFTER THE ECLIPSE – the opposite situation, where the observer experiences a deep partial phase, but the remote object observed is already much more illuminated by a thicker solar crescent. Likewise, the previous model of the incident radiance varied continuously across the line of sight (Pic. 15). In this case, the object is less visible due to the fainter contrast between it and the horizon sky (Pic. 16). Moreover, the light scattering on atmospheric particles plays a bigger role due to the higher level of illumination. Both with this and the previous model I have assumed that the lunar shadow comes from the right according to my own observation manner.
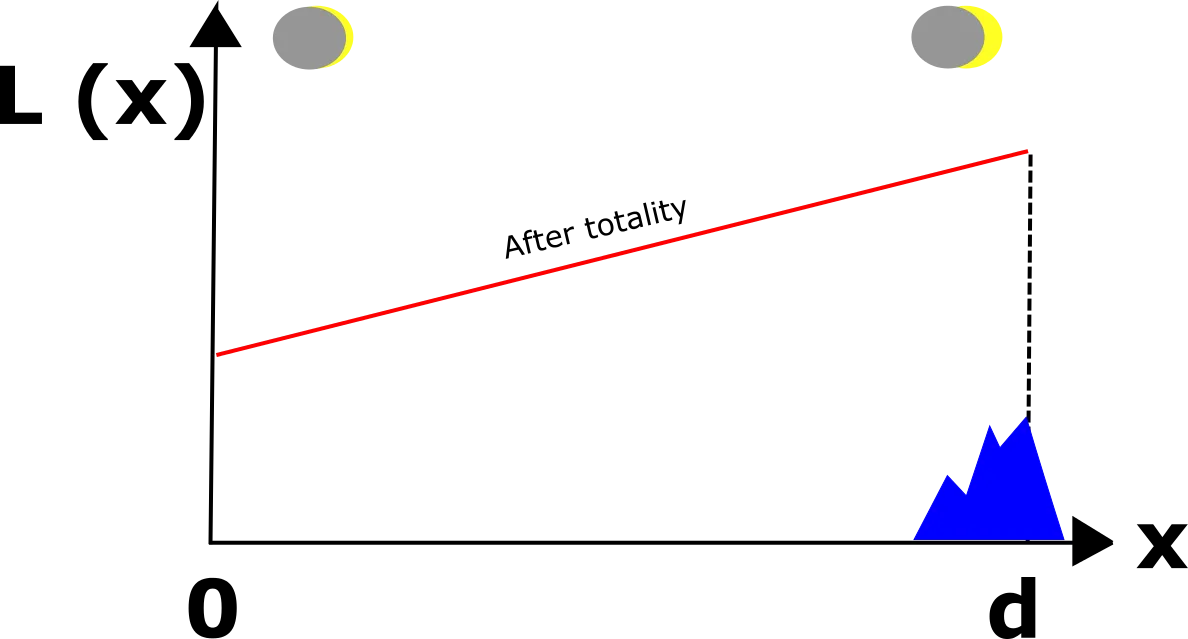
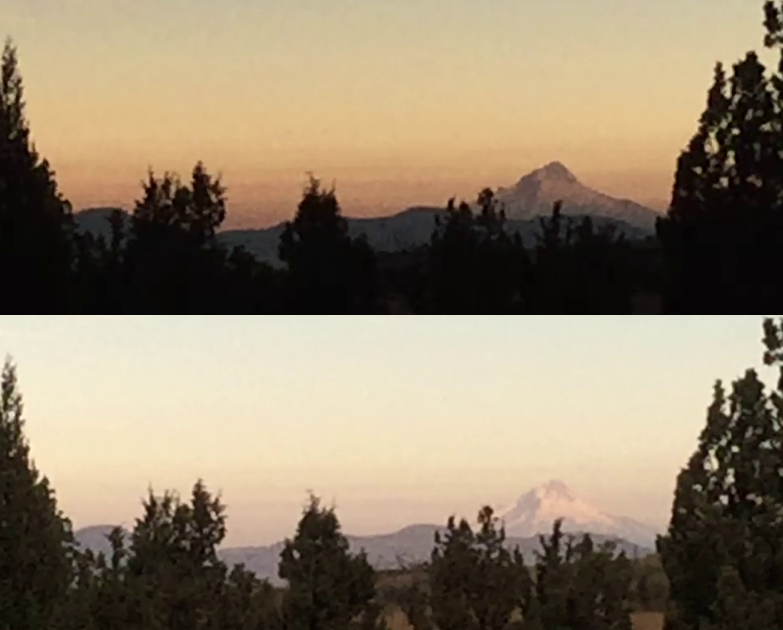
G. HETEROGENEOUS IRRADIATION CAUSED BY NONUNIFORM CLOUD COVER – analog to total solar eclipse situation, but on a smaller scale, what is roughly described in my previous article. Bigger irradiation is to be seen locally alongside the line of sight as well as the shadowed area. Due to this situation, even on a hazy day, the visibility range appears to be longer than under a clear sky.
The illumination models, that refer to a penumbral case are new to me, I was considering only the first 4 after the eclipse and 5th one earlier for long-distance observation considerations. The Moon’s shadow velocity was 839m/s. The typical penumbral radius is greater than 3000km, whereas the umbra diameter is only of the order of 100 km (Vollmer, Shaw, 2018). The illuminance changes during the solar eclipse occur along with the logarithmic function, but in order to better understand, this phenomenon will be easier to simplify it by a simple case of step-wise function as per below (Vollmer, Shaw, 2018). This plot shows the lunar shadow coming from left to right as per my own observation of the Owl Creek Mts.
5. EFFECT OF VISUAL RANGE CHANGES DURING SOLAR ECLIPSE
The visual range changes during the total solar eclipse and can be visible once the shadow length is longer than the critical value, which depends on many factors like haze concentration, distance to the object, Sun position in the sky, etc. The penumbral diameter is much larger than any practical visual range. Thus for these short distances, any changes in illuminance and radiance may be approximated as a linear function for most eclipses. As a result, the observed effect of visual range changes is pretty much the same when looking directly toward the shadow-in and shadow-out sky.
The situation can be more complicated when we are considering a deep partial and total phase of the eclipse. Then you can spot, that the visual range varies according to logarithmic function behavior reaching the maximum value in the shadowed area. While the shadow of the Moon is racing above the observer’s head, the landscape looks like civil twilight. At this moment a visual range is the best and is eventually restricted by the edge of the umbra. Once the visual range under normal daytime conditions is shorter due to haze concentration the lack of direct solar radiation can extend it significantly up to the critical moment. This critical moment will occur when a far dark-looking object merges with a shaded haze attenuating a bright skylight beyond. As we know from the previous section, an umbral region is not only one place with an extended visual range (Pic. 16). The difference in light scattering by haze particles between the umbral and non-umbral regions is huge, however, the visual range starts to extend already at deep partial phase of the eclipse, when a direct illumination diminishes (Pic. 17, 18). An analog situation takes place at the most extreme moments of totality, where the umbral edge is close enough to let the diffused radiated skylight come through the umbral region and make the haze particles slightly brighter (Pic. 17).
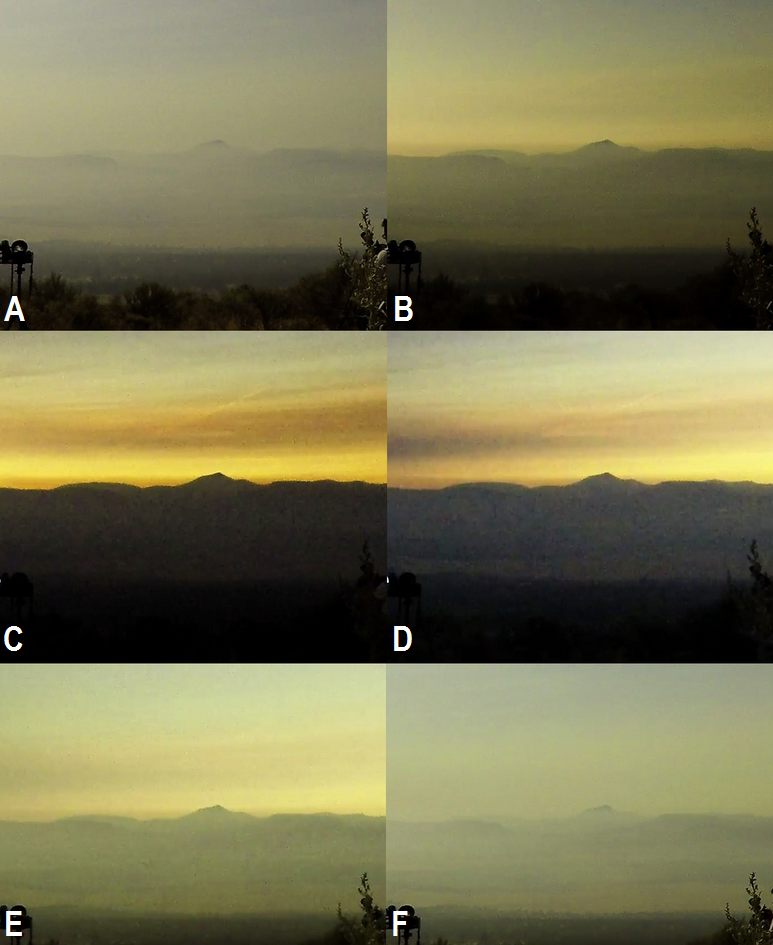
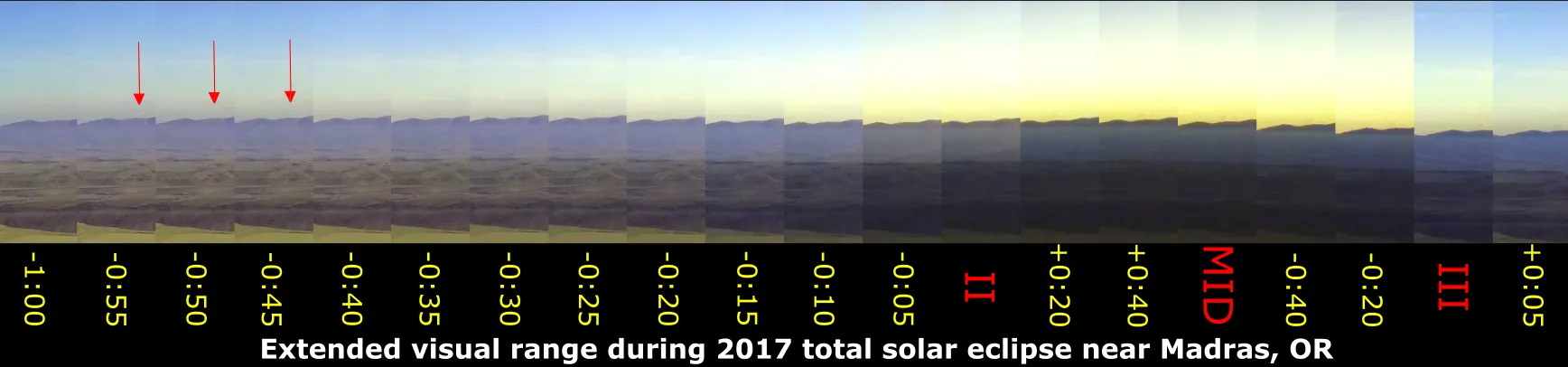
Usually, the biggest extension of visual range during the partial solar eclipse takes place when obscuration is bigger than 98% and increments likewise logarithmic function up to umbral contact. In the images provided (Pic. 17, 18) we can see, that mountains became better visible (or at least visible) at about 1 min before totality when solar obscuration was about 98,8%. These sequences show quite a big illumination and visual range difference, that happens during this moment, but smaller changes could be visible a bit earlier.
The extremely fast umbra makes a total solar eclipse a very dynamic phenomenon. As a consequence the visibility circumstances are fleeting. In order to mark these changes is good to show the plots, that refer to a particular situation (Pic. 19, 20). The distance from the observer to the edge of the umbra determines the degree of the distant-sky airlight attenuation. This attenuation changes every second as the umbra moves. It can be seen in the footage, how remote objects slightly change the tint due to this. From a practical point of view, the smallest sky airlight attenuation is observed at the beginning of the end of totality at the distant object depending on where we are looking (Pic. 19, 20). After the totality, the radiance in the penumbra starts to increase linearly between the observer and object. The horizon radiance has an additional distant-sky radiance from the other penumbra observed above the horizon seen in the distance. The contrast between the remote object observed and the horizon sky evaluates throughout a whole eclipse event from the moment when the penumbra touches it until the 4th contact occurring at the observation place. To reaffirm the result of this observation is good to observe more objects at once, because one object, that contrast alone may not be sufficient. Next to the horizon and objects, mountains located far away a key role plays also the horizon sky’s brightness. Over the course of the eclipse phenomena, the horizon sky brightness changes remain asymmetrical, which will be raised over the course of the next articles. Nevertheless here is an occasion to explain again this issue in order to visibility conditions. The horizon sky brightness, as well as a whole illuminance, decreases by more than four orders of magnitude. Because this process is asymmetrical for example shadow-in side of the eye does not receive enough radiation at the beginning of the eclipse the mountains theoretically cannot be detected. Practically contrast between the dark horizon and bright sky alright located behind the opposite part of the shadow will allow it. At this moment a shadow-out sky will offer a faint, but better view of remote mountains.
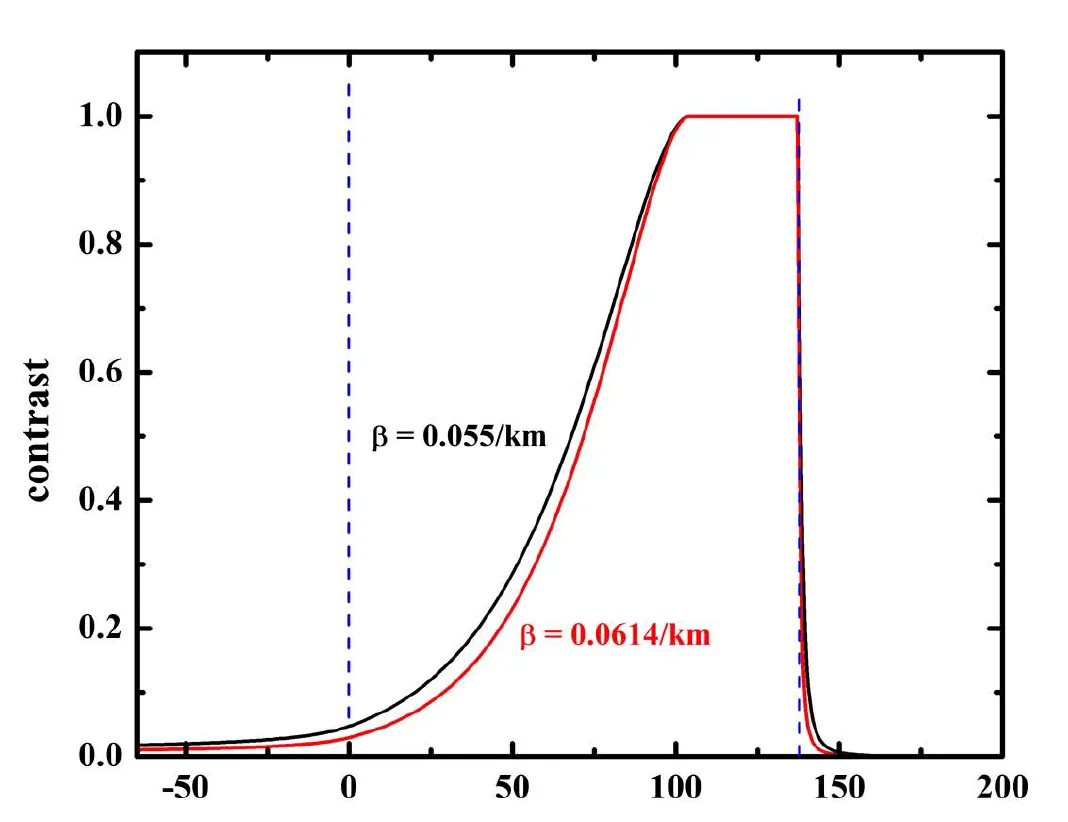
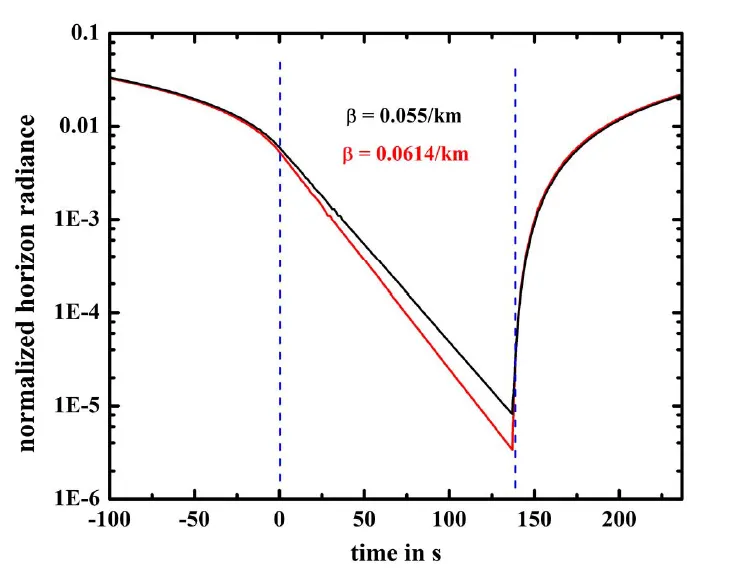
From a practical point of view, the lowest shadow-in section of horizon radiance occurs always right after the beginning of the eclipse. Conversely, as the above plot (Pic. 20) shows, the shadow-out horizon radiance is the lowest just before the end of the eclipse. This is the result of the lunar shadow moving across the sky, whereas one section of the sky is getting brighter and another one is getting darker in the same moment of the eclipse. Following the plot above (Pic. 20), before the totality, the horizon radiance drops slowly, but quicker in the totality window. A shadow-out horizon sky scatters less amount of light due to the umbral approaching. Finally, when the umbra covers a vast majority of the sky, as it happens during a typical 2-3 minutes-length totality the horizon radiance is faint enough to remain in civil twilight-looking conditions. Because the most important part of the atmosphere, which diffuses a lot of light is the stratosphere (ozonosphere), then even during the longest total solar eclipses there is no option to engulf this whole horizon sky by lunar shadow (as long as we are talking about a zenithal type of eclipse, unlike to horizontal ones, where shadow cone will look like a path dividing the sky for two separate bright sections, about which I will write in the future). In the outcome, the horizon sky at the opposite side of the umbra will be illuminated and bright enough to make a decent contrast with a distant object being shaded by the umbra. The exception to this can be an extremely thick haze layer, which will tend to attenuate this light.
The moment, where the shadow-out horizon brightness is the lowest usually coincides with the end of the eclipse. Right after this moment, for the next 30-40 seconds, the horizontal brightness dramatically increases, which is shown in the chart above (Pic. 20). This is the moment of the solar eclipse light transition when the Baily’s Beads sunlight starts to beat up the light scattered at the horizon sky, drowning it out eventually from the brighter, upper section of sky. The distant objects become much less visible, or even invisible to the observer. Instead of this from the already illuminated observation point, an interesting grey shadow cone is to be visible. I will back to this issue in a future article, fully designated for the lunar shadow movement during the totality.
To summarize this section I would like to bring good examples of the visual range difference between total eclipse and non-eclipse or at least partial eclipse conditions.
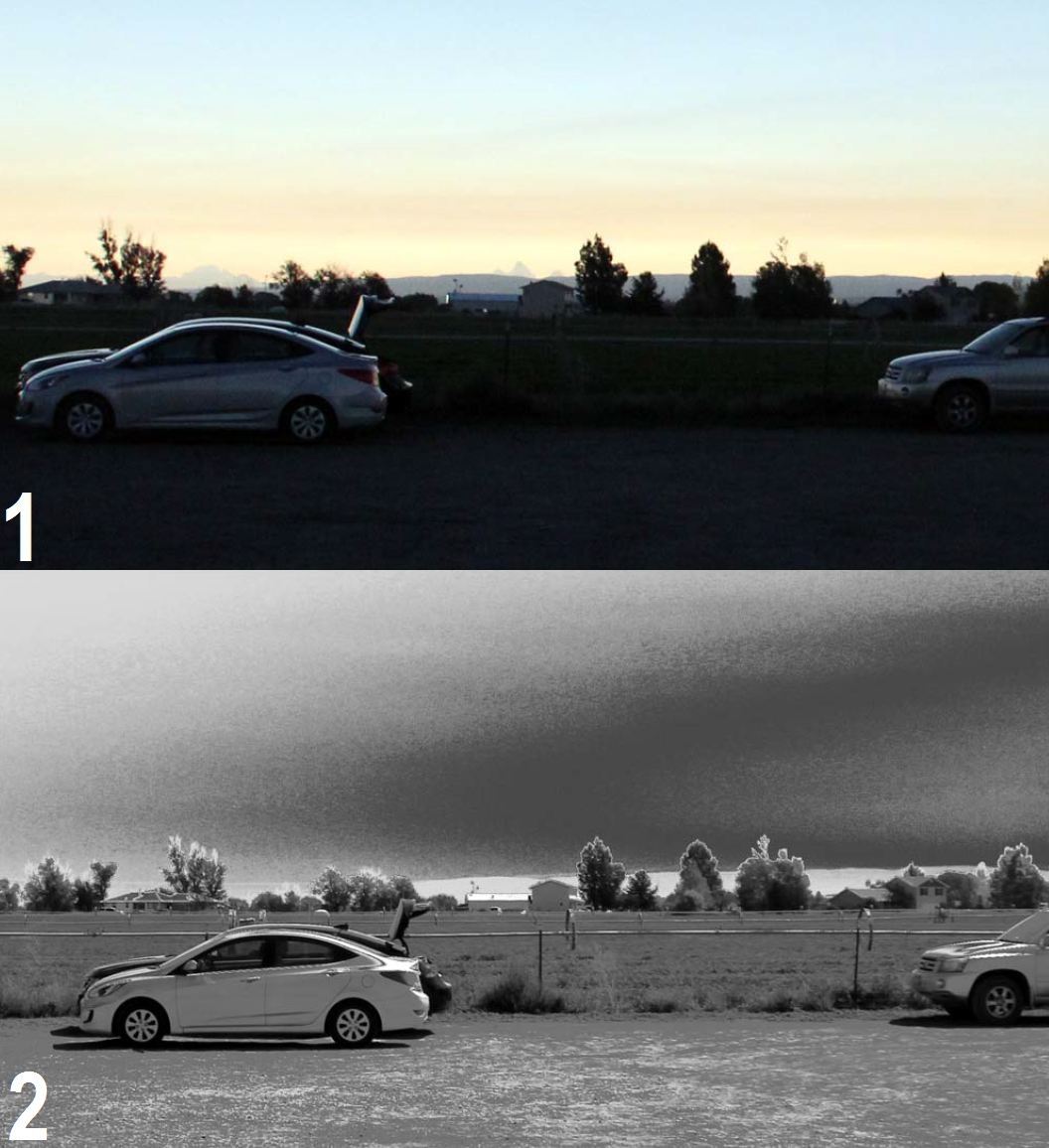
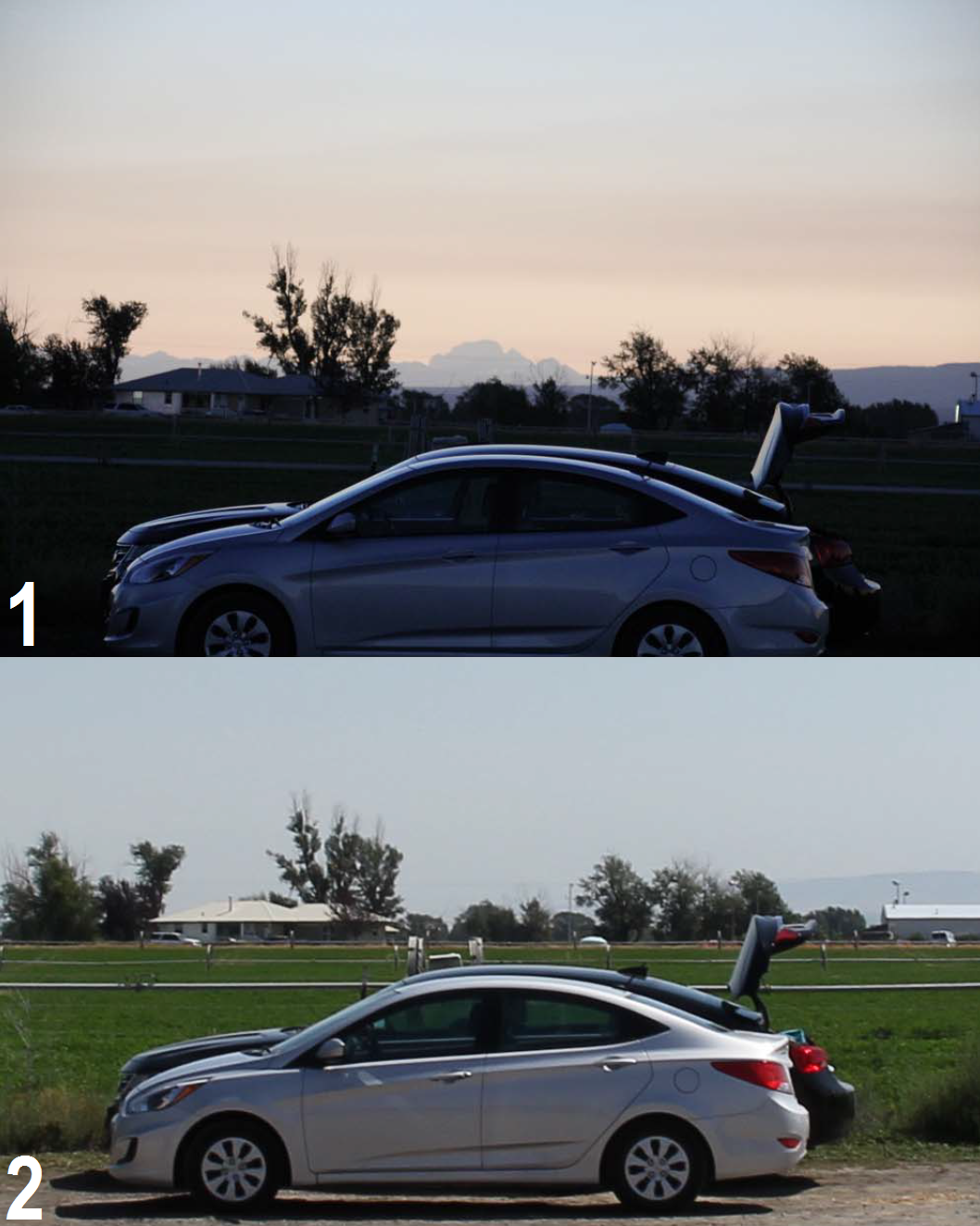
Now I would like to show what I spot from my observation venue. I was looking towards the Owl Creek Mts. located 35-65 km ahead (Pic. 23). To my surprise, I could spot even distant Wind River Range, located about 90 km ahead! (Pic. 24).
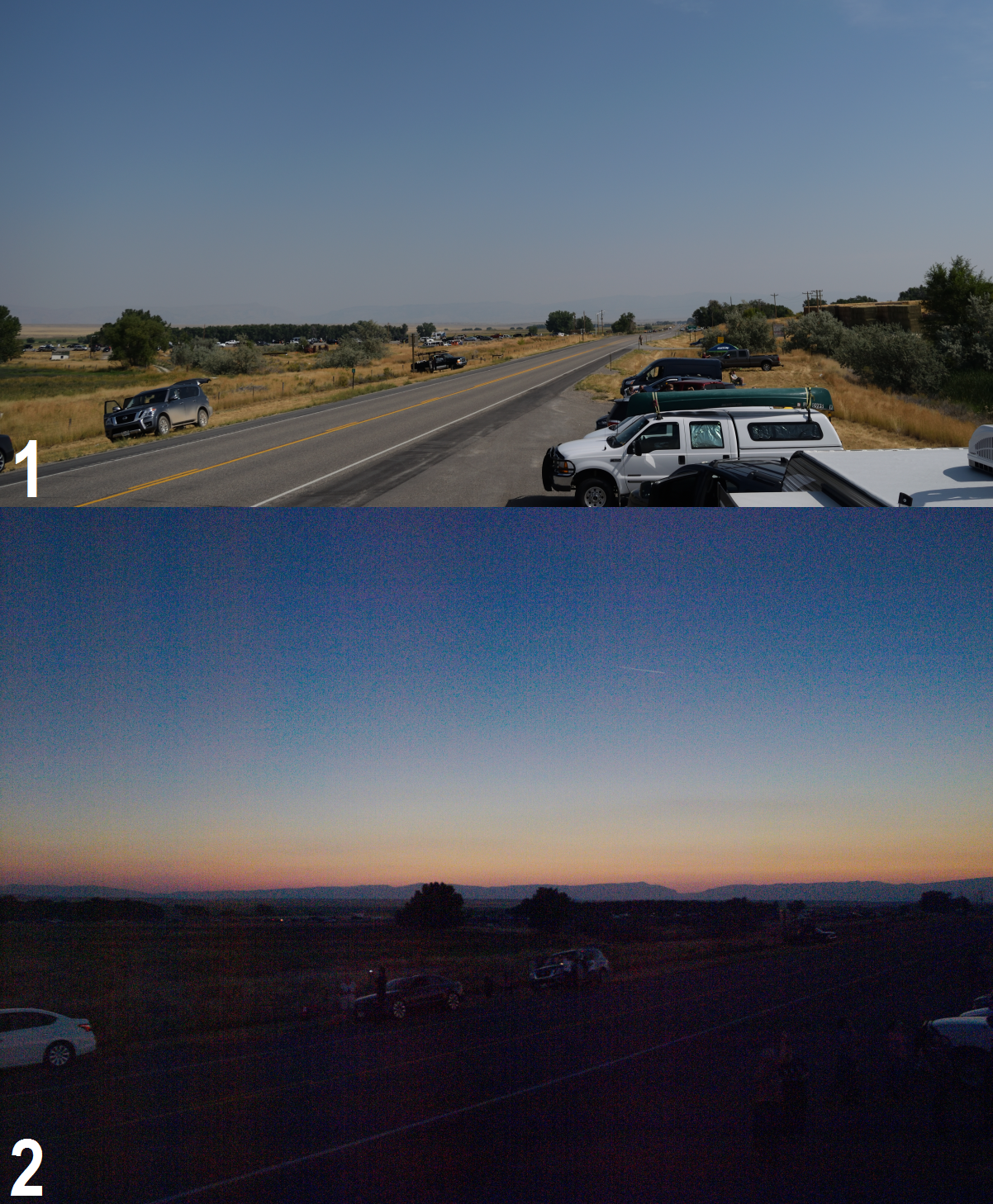
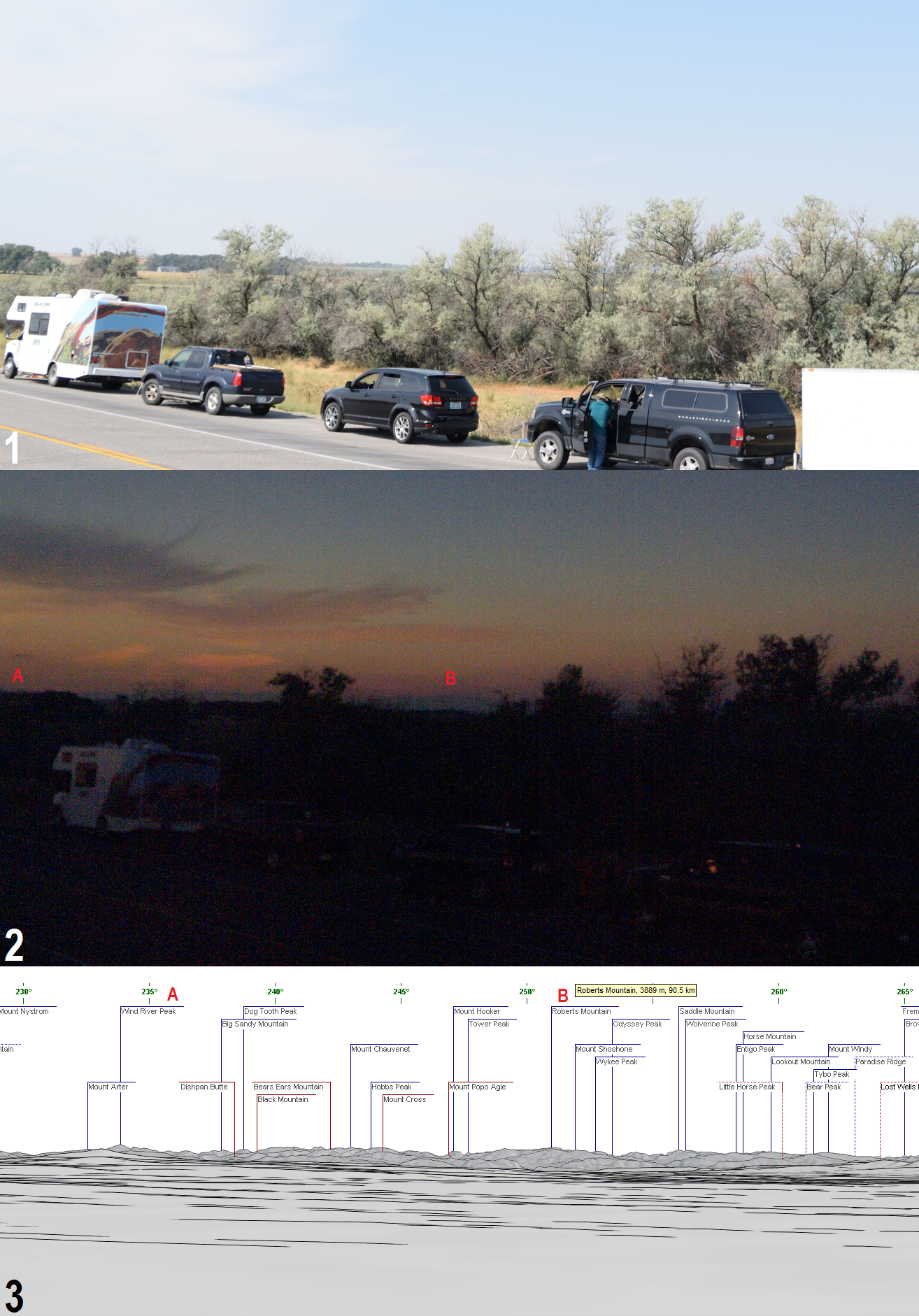
The Wind River range started to be visible about 40-30 sec before totality (Pic. 25) and just before the solar eclipse light transition.
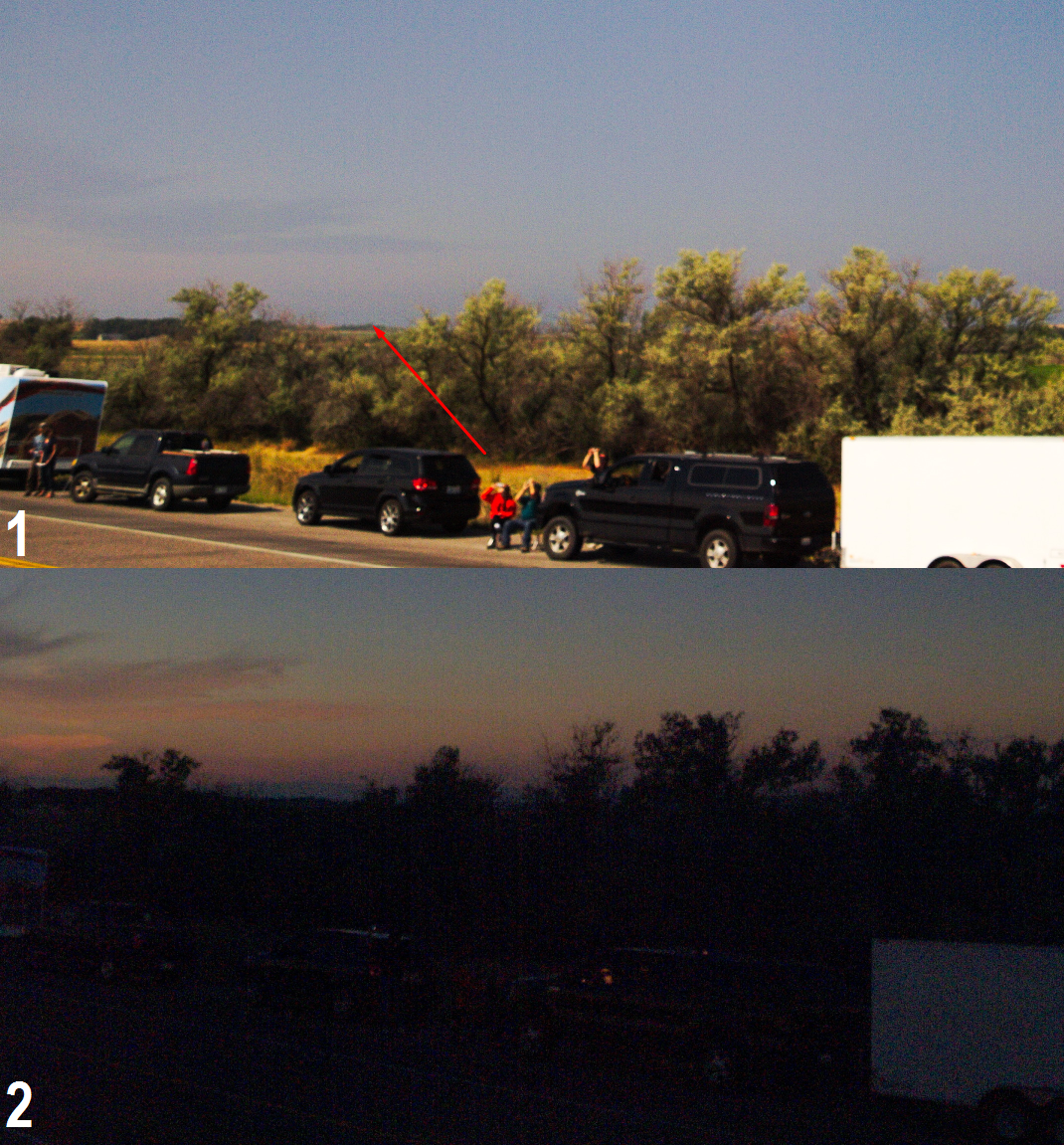
In the end, is very important to say about another issue, that can sometimes play a major role in the visual range during the totality. This is cloudiness. Clouds behave like any other object, shaded during the eclipse. Despite their white surface clouds appear to look black against a much brighter horizon sky. This is the same mechanism, as we can see before sunrise or after sunset when the Sun is deep enough and doesn’t illuminate the clouds directly. In this case, the visual range is reduced dramatically (Pic. 26) in places, that have been described here.
It doesn’t work for high-level clouds, which usually during the total solar eclipse remain illuminated just above the horizon (Pic. 26) as long as this is a zenithal type of eclipse.
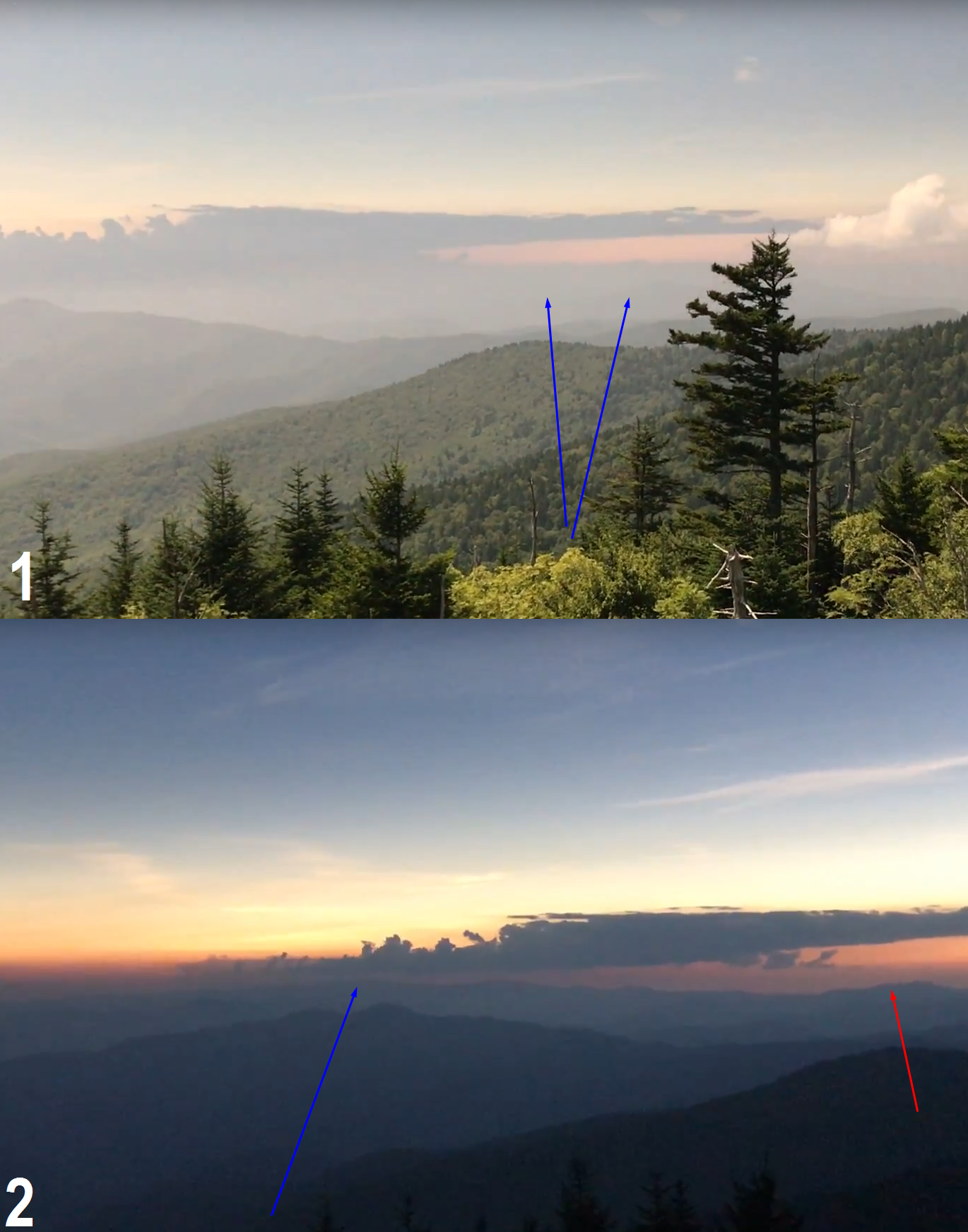
6. CONCLUSIONS
I have shown fairly incidentally the observation of extended visual range during the Great American Eclipse. Most of the things shown in this article have been analyzed from photos and footage because I didn’t spot this phenomenon directly during the eclipse. Nonetheless gathering the data from a few different observation circumstances under a different level of haze combined with probably the first scientific article about it allowed me to make this wide description. Due to overheating focus on solar corona, only the visibility changes phenomena during the totality remains out of common interest. The umbra presence causes a visual range extension even on tens of kilometers during the totality under hazy conditions. The hazy skies reduce the visual range outside the totality. These simple models covered in this article show examples of different situations, that are to be seen around the totality. Some situations are analog also to the deep partial solar eclipses, where umbra location lies at least at the equal distance to visibility range or at least for obscuration higher than 86-88%, where the penumbral affecting on visibility towards the shadow-in sky is discernable. It would be interesting to conduct further experiments during future eclipses to enable further refinement and validation of the results of this observation, especially since every totality is different. The pivot of this kind of observation is positioning a camera to view distant mountains, that are not visible in regular daylight (Vollmer, Shaw, 2018). For this purpose, the Urlich Deuschle and PeakFinder mountain panorama generator will be helpful. Doing this observation during an annular eclipse would be an interesting extension of this issue, especially when we use time-lapse or continuous video techniques in order to record a fascinating view of the changing contrast.
Mariusz Krukar
References:
- Koonen G.P., Hinz C., 2008, Visibility of stars, halos and rainbows during solar eclipses, (in:) Applied Optics, vol, 47 i.34, p.H14-H24
- Vollmer M., Shaw J.A., 2018, Extended visual range during solar eclipses, (in:) Applied Optics, vol. 57, no12 p. 3250-3259
Links:
- Urlich Deuschle panorama generator
- https://www.peakfinder.org/
- Full description of this observation with large images
Youtube:
Read also:
- First time in the shadow
- Total solar eclipse vizualisation on Google Earth, part 1
- Horizontal visibility as a main factor of long-distance observations, part 1 – weather, astronomical and optical effects